Chemical science continues to drive groundbreaking progress in industries like pharmaceuticals and materials development. One remarkable technique leading this evolution is allyl-thiol click on chemical post-modification IR, a process that combines precision chemistry with advanced analytical tools. This approach enables scientists to modify molecules with unparalleled accuracy, enhancing their properties for a wide range of applications.
Click chemistry has become an essential tool in modern research, streamlining the creation of complex molecular structures. The allyl-thiol click on chemical post-modification IR method excels in delivering targeted molecular changes after synthesis. Its efficiency and adaptability allow researchers to enhance the functionality of molecules, making them suitable for highly specific industrial and scientific uses.
A key component of this method is infrared (IR) spectroscopy, which provides a non-invasive, highly sensitive way to monitor and evaluate chemical transformations. Through allyl-thiol click on chemical post-modification IR, scientists gain real-time insights into reaction progress, ensuring precision and reliability. IR spectroscopy also plays a crucial role in verifying product quality and consistency, making it an indispensable part of the process.
The integration of allyl-thiol click on chemical post-modification IR is transforming how researchers approach molecular customization. By merging efficient chemistry with cutting-edge analytical techniques, this method empowers industries to innovate with greater precision, reliability, and speed. As a result, it is driving advancements in developing novel materials and compounds tailored to meet the demands of modern technology.
What Is Allyl-Thiol Click Chemistry?
Allyl-thiol click chemistry refers to a highly specific reaction in which an allyl group—a simple organic structure with a reactive double bond—combines with a thiol group (-SH) to form a stable covalent bond. This reaction is characterized by its rapid completion, minimal byproduct formation, and adaptability under mild conditions. Unlike traditional chemical processes that may involve complex reagents or harsh environments, allyl-thiol click chemistry operates efficiently without requiring elevated temperatures or aggressive solvents.
The foundation of click chemistry as a concept, pioneered in the early 21st century, emphasized simplicity and reliability. Allyl-thiol reactions epitomize these principles by offering a streamlined path to creating functionalized molecules with diverse applications. This method has gained traction due to its compatibility with a wide range of substrates, enabling researchers to tailor molecules for specific functionalities, particularly in post-modification processes.
Mechanistic Insights into Allyl-Thiol Click Chemistry
The allyl-thiol click reaction is a versatile and robust chemical transformation that leverages the reactivity between allyl groups and thiols. This reaction can proceed through different mechanistic pathways, each offering unique advantages depending on the reaction conditions.
- Radical Pathway: In the radical mechanism, thiol groups (-SH) are activated into reactive thiyl radicals using radical initiators like azobisisobutyronitrile (AIBN) or exposure to UV light. These thiyl radicals then attack the allyl double bond, leading to the formation of a carbon-centered radical intermediate. This intermediate undergoes propagation, forming a stable thioether bond. This pathway is especially advantageous in polymer chemistry and surface functionalization, where uniform reactivity across substrates is critical.
- Nucleophilic Pathway: Alternatively, a base such as triethylamine (TEA) or an alkoxide can deprotonate the thiol group to generate a nucleophilic thiolate ion. This species readily reacts with the allyl double bond to form the desired thioether linkage. The nucleophilic approach is preferred for reactions requiring mild conditions or those involving sensitive substrates, such as biomolecules.
Understanding the mechanistic basis is crucial for optimizing the selectivity and efficiency of allyl-thiol click chemistry, particularly when applied to complex systems.
The Role of Allyl-Thiol Reactions in Chemical Post-Modification
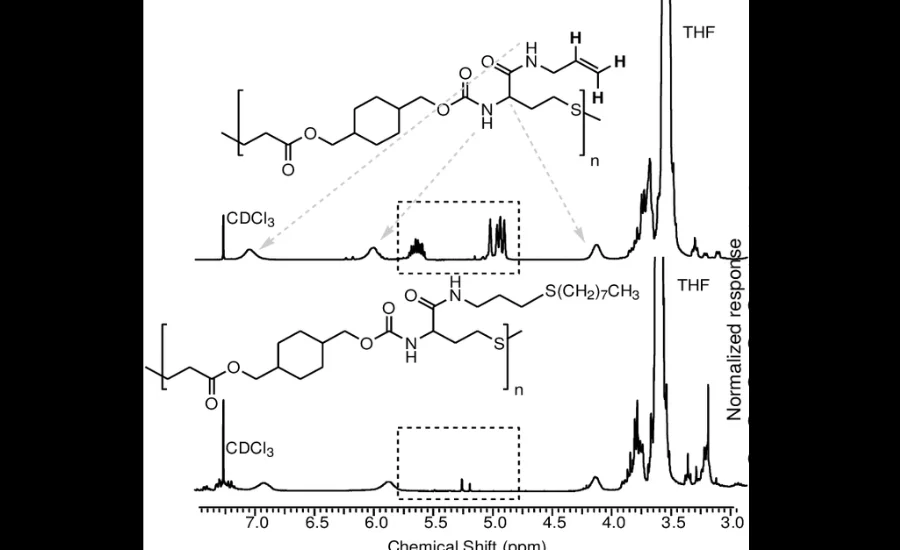
Chemical post-modification refers to the alteration of a molecule or material after its initial synthesis to enhance or customize its properties. Allyl-thiol click reactions have emerged as a cornerstone of this approach due to their precision and reliability. By leveraging these reactions, scientists can introduce functional groups or modify molecular structures to meet specific design goals.
For example, in pharmaceuticals, post-modification through allyl-thiol reactions can improve a drug’s solubility, stability, or targeted delivery capabilities. Similarly, in materials science, polymers and nanoparticles can be functionalized to exhibit properties such as increased durability, tailored surface interactions, or responsiveness to environmental triggers. These modifications, achieved through allyl-thiol click chemistry, offer a level of control and efficiency that is difficult to achieve with other methods.
Catalyst Selection and Reaction Optimization
The efficiency of the allyl-thiol reaction depends heavily on the choice of catalysts and reaction parameters. Catalysts play a pivotal role in controlling reaction rates, minimizing side products, and enabling mild reaction conditions.
- Metal Catalysts: Transition metals such as palladium, platinum, and gold have been employed to catalyze allyl-thiol reactions. These catalysts enable reactions under ambient conditions and often increase the reaction rate. However, the use of metals may pose challenges in applications requiring biocompatibility.
- Organic Catalysts: Bases like imidazoles and other organocatalysts are attractive alternatives, offering green and sustainable pathways. They also provide excellent selectivity and are less likely to interfere with sensitive functional groups.
- Photocatalysis: Light-activated catalysts or direct UV light can be used to initiate allyl-thiol reactions. Photocatalysis allows for precise control over the reaction, enabling spatiotemporal tuning. This is particularly valuable in applications like patterned surface functionalization.
Key parameters such as reaction temperature, solvent choice, and thiol-to-allyl ratio must be carefully optimized. By adjusting these factors, researchers can enhance yields, reduce reaction times, and tailor the process to specific substrates or applications.
Advantages of Allyl-Thiol Click Chemistry
The advantages of allyl-thiol click chemistry extend beyond its efficiency. This method is celebrated for its high selectivity, as it minimizes unwanted side reactions and ensures that the desired product is obtained in high yields. Additionally, the mild conditions under which these reactions take place make them suitable for sensitive molecules that might degrade under harsher environments.
Moreover, allyl-thiol reactions are environmentally friendly, often conducted in aqueous media without the need for toxic reagents. This aligns with the principles of green chemistry, making the technique increasingly attractive for industrial applications where sustainability is a priority.
Infrared Spectroscopy in Post-Modification
IR spectroscopy has become an indispensable tool in the field of chemical modification, particularly when combined with allyl-thiol click chemistry. By analyzing how molecules absorb infrared light, IR spectroscopy provides detailed information about the functional groups and chemical bonds within a sample. This makes it ideal for monitoring the progress of post-modification reactions and verifying the success of molecular alterations.
For instance, during an allyl-thiol reaction, the emergence or disappearance of specific absorption bands in the IR spectrum can indicate the formation of new bonds or the consumption of reactants. This enables researchers to optimize reaction conditions in real-time, ensuring the highest efficiency and accuracy in their work.
Applications of Allyl-Thiol Click Chemistry in Industry
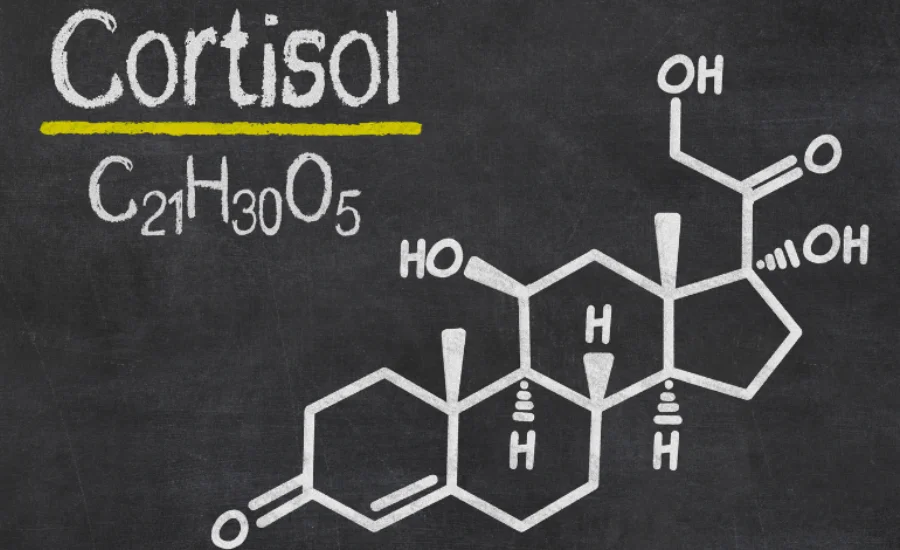
The integration of allyl-thiol click chemistry with post-modification processes has transformed a variety of industries. In the pharmaceutical sector, this method is used to create advanced drug delivery systems. By modifying biomolecules with allyl-thiol reactions, researchers can design drugs that specifically target diseased tissues, reducing side effects and improving therapeutic outcomes.
In materials science, allyl-thiol click chemistry plays a vital role in enhancing the properties of polymers and other materials. For example, introducing thiol-functionalized groups into polymer chains can improve adhesion, flexibility, or resistance to environmental degradation. Similarly, in nanotechnology, functionalized nanoparticles created through allyl-thiol reactions are used in applications ranging from diagnostics to smart materials that respond to external stimuli.
Emerging Trends and Innovations
- Photoinitiated Reactions: Light-driven allyl-thiol reactions are gaining traction for their precision and environmental friendliness. By utilizing visible light, these reactions eliminate the need for toxic catalysts.
- Dual-Click Strategies: Coupling allyl-thiol click chemistry with other click reactions, such as thiol-ene or azide-alkyne cycloaddition, is paving the way for multi-functionalized materials with tailored properties.
- Self-Healing Materials: Incorporating allyl-thiol chemistry into dynamic polymer networks has enabled the creation of self-healing materials. These materials autonomously repair damages, making them ideal for long-term and high-performance applications.
- Biomedical Innovations: In biomedicine, allyl-thiol reactions are being explored for site-specific drug delivery systems, where functionalized nanocarriers can respond to external stimuli for targeted release.
Functional Group Compatibility
One of the defining strengths of allyl-thiol click chemistry is its exceptional functional group tolerance. This compatibility makes it a preferred choice for modifying complex molecules and materials.
- Tolerance for Polar Groups: Hydroxyl (-OH), carboxylic acid (-COOH), and amino (-NH₂) groups remain unaffected during the reaction, allowing for versatile modifications without altering the substrate’s inherent properties.
- Stability of Sensitive Bonds: Under mild reaction conditions, ester, amide, and ether linkages are preserved, ensuring that the structural integrity of polymers, proteins, or other delicate systems is maintained.
- Reactivity with Aromatics: Allyl groups on aromatic systems are highly reactive, even in the presence of electron-withdrawing or donating substituents. This widens the scope of substrates that can undergo allyl-thiol modification.
This wide-ranging compatibility enables allyl-thiol click chemistry to address the needs of diverse fields, from medicinal chemistry to materials science.
Role of Infrared (IR) Spectroscopy
Infrared spectroscopy is an essential tool for monitoring the allyl-thiol click reaction. It provides real-time insights into bond formation and reaction progress.
Key Vibrational Modes:
- Allyl C=C Stretching: The allyl group exhibits characteristic IR absorption at 1640–1680 cm⁻¹, which diminishes as the reaction proceeds.
- Thiol S-H Stretching: Thiols show a distinct band around 2550–2600 cm⁻¹. Disappearance of this band confirms the consumption of thiol groups.
- Thioether C-S Stretching: Newly formed thioether bonds produce vibrations near 700–800 cm⁻¹, indicating successful bond formation.
- ATR-IR (Attenuated Total Reflectance): This technique is particularly useful for monitoring reactions on solid surfaces or in heterogeneous systems.
- 2D-IR Spectroscopy: By capturing dynamic molecular interactions, 2D-IR can provide detailed kinetic information, aiding in reaction optimization.
Advantages and Limitations
Advantages
- High Efficiency: The reaction is fast and produces minimal side products, making it ideal for high-throughput applications.
- Mild Conditions: The reaction is compatible with temperature-sensitive and biologically active molecules.
- Broad Compatibility: Functional groups like esters, amides, and hydroxyls remain unaltered, expanding the range of usable substrates.
Limitations
- Oxidation Sensitivity: Thiols are prone to oxidation, which may reduce reaction efficiency if not properly handled.
- Metal Residues: When metal catalysts are used, purification steps may be necessary to remove residual metals.
- Limited Reversibility: Once formed, thioether bonds are stable, making the reaction challenging to reverse or modify post-synthesis.
Challenges and Innovations
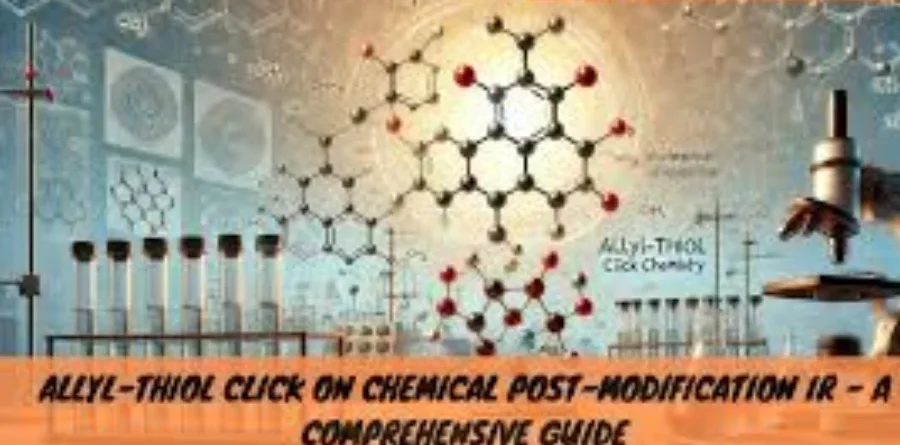
While allyl-thiol click chemistry offers numerous advantages, it is not without challenges. Achieving complete conversion of reactants can sometimes be difficult, especially when dealing with complex or sterically hindered molecules. Additionally, optimizing reaction conditions to ensure maximum efficiency and selectivity requires careful consideration of factors such as temperature, solvent, and catalyst choice.
Despite these challenges, ongoing innovations in the field are addressing these issues. Researchers are developing new catalysts and reaction protocols to expand the scope of allyl-thiol click chemistry, making it more robust and versatile. Advances in IR spectroscopy, including the development of more sensitive and portable instruments, are also enhancing the ability to monitor and analyze these reactions with greater precision.
Allyl-thiol click on chemical post-modification ir’s Future
As the demand for sustainable and efficient chemical processes grows, the role of allyl-thiol click chemistry is poised to expand. Future research is likely to focus on refining this technique for even broader applications, such as in personalized medicine, where tailored molecular modifications can enable customized therapies.
In addition, the integration of allyl-thiol reactions with emerging technologies, such as artificial intelligence and machine learning, could revolutionize the way these processes are optimized. By analyzing large datasets from IR spectroscopy and other analytical techniques, algorithms could identify patterns and recommend the best reaction conditions for specific applications.
Final Words
Allyl-thiol click on chemical post-modification IR is revolutionizing molecular design by combining precision chemistry with advanced analytical tools. This method utilizes the highly selective allyl-thiol click reaction to introduce targeted modifications to molecules, enhancing their properties for applications in pharmaceuticals, materials science, and beyond.
Key to this process is infrared (IR) spectroscopy, which provides real-time insights into reaction progress. By monitoring functional group changes, IR spectroscopy ensures unparalleled accuracy and efficiency in molecular transformations. This makes it indispensable for tailoring molecules to specific industrial or scientific needs.
The method’s compatibility with diverse functional groups, eco-friendly conditions, and scalability underscores its importance in advancing green chemistry principles. Applications range from improving drug delivery systems to creating responsive materials and self-healing polymers.
As innovations like photoinitiation and dual-click strategies emerge, allyl-thiol click on chemical post-modification IR will continue to drive breakthroughs, empowering industries with tools for sustainable and precise molecular engineering.
For cutting-edge insights into chemical science and its transformative applications, explore the innovations driving industries forward at Insight Rays.